1. Rahmer J, B rnert P, Groen J, Bos C. Three-dimensional radial ultrashort echo-time imaging with T2 adapted sampling. Magn Reson Med, 2006 May;55:1075-1082. doi.org/10.1002/mrm.20868.
2. Du J, Bydder M, Takahashi AM, Carl M, Chung CB, Bydder GM. Short T2 contrast with three-dimensional ultrashort echo time imaging. Magn Reson Imaging, 2011 May;29(4):470-482. doi.org/10.1016/j.mri.2010.11.003.
3. Weiger M, W u M, Wurnig MC, et al. ZTE imaging with long-T2 suppression. NMR Biomed. 2015 Feb;28(2):247– 254. doi.org/10.1002/nbm.3246.
4. Li C, Magland JF, Rad HS, Song HK, Wehrli FW. Comparison of optimized soft-tissue suppression schemes for ultrashort echo time MRI. Magn Reson Med 2012 Sep;68(3):680-689. doi.org/10.1002/mrm.23267.
Figure 1.
T2 decay of short T2 species such as cortical bone. Conventional imaging technique has readout time that occurs when the signal has significantly decayed. ZTE or UTE imaging are needed to capture these signals.
A
Figure 2.
Comparison of bone imaging using conventional MR, UTE with echo subtraction/IR, and ZTE acquisition. (A) Conventional MR, no signal with long TE to determine bone from void. (B) UTE or ZTE with long T2 suppression using inversion recovery or echo suppression provides direct imaging of the bone, however, with very low SNR. (C) ZTE/UTE demonstrates signal in bone with a flat contrast in the surrounding tissue enabling differentiation between air and soft tissue.
B
Figure 2.
Comparison of bone imaging using conventional MR, UTE with echo subtraction/IR, and ZTE acquisition. (A) Conventional MR, no signal with long TE to determine bone from void. (B) UTE or ZTE with long T2 suppression using inversion recovery or echo suppression provides direct imaging of the bone, however, with very low SNR. (C) ZTE/UTE demonstrates signal in bone with a flat contrast in the surrounding tissue enabling differentiation between air and soft tissue.
C
Figure 2.
Comparison of bone imaging using conventional MR, UTE with echo subtraction/IR, and ZTE acquisition. (A) Conventional MR, no signal with long TE to determine bone from void. (B) UTE or ZTE with long T2 suppression using inversion recovery or echo suppression provides direct imaging of the bone, however, with very low SNR. (C) ZTE/UTE demonstrates signal in bone with a flat contrast in the surrounding tissue enabling differentiation between air and soft tissue.
5. Robson M, Gatehouse PD, Bydder M, Bydder GM. Magnetic Resonance: An Introduction to Ultrashort TE (UTE) Imaging. J Comput Assist Tomogr. 2003, Nov-Dec;27(6):825-846. doi.org/10.1097/00004728-200311000-00001.
6. Gatehouse PD, Bydder GM. Magnetic Resonance Imaging of Short T2 Components in Tissue, Clin Radiol. 2003 Jan;58(1):1-19, doi.org/10.1053/crad.2003.1157.
7. Carl M, Bydder GM, Du J. UTE imaging with simultaneous water and fat signal suppression using a time-efficient multispoke inversion recovery pulse sequence. Magn Reson Med. 2016 Aug;76(2):577-582. doi.org/10.1002/mrm.25823.
8. Wiesinger F, Bylund M, Yang J, et al. Zero TE-based pseudo-CT image conversion in the head and its application in PET/MR attenuation correction and MR-guided radiation therapy planning. Magn Reson Med. 2018 Oct;80(4):1440–1451. doi.org/10.1002/mrm.27134.
Figure 3.
oZTEo pulse sequence and 3D radial readout trajectory.
8. Wiesinger F, Bylund M, Yang J, et al. Zero TE-based pseudo-CT image conversion in the head and its application in PET/MR attenuation correction and MR-guided radiation therapy planning. Magn Reson Med. 2018 Oct;80(4):1440–1451. doi.org/10.1002/mrm.27134.
A
Figure 4.
(A) Volume rendering using Volume Illumination feature in GE’s AW Volume Viewer. (B) Results can also be exported to common 3D printing formats, such as STL.
B
Figure 4.
(A) Volume rendering using Volume Illumination feature in GE’s AW Volume Viewer. (B) Results can also be exported to common 3D printing formats, such as STL.
A
Figure 6.
Effect of readout bandwidth. Increasing BW can reduce chemical shift artifact and T2 contrast in the background. However, if BW is too high, blurring will occur. (A) BW = +/-62 kHz shows high SNR but with chemical shift artifact. (B) BW = +/-83 kHz shows reduced chemical shift as BW increases. (C) BW = +/-100 kHz shows blurred boundary that may occur if BW is too high. Images acquired at 3.0T, 1.2 x 1.2 x 1.2 mm, NEX 4.5, 3:47 min.
B
Figure 6.
Effect of readout bandwidth. Increasing BW can reduce chemical shift artifact and T2 contrast in the background. However, if BW is too high, blurring will occur. (A) BW = +/-62 kHz shows high SNR but with chemical shift artifact. (B) BW = +/-83 kHz shows reduced chemical shift as BW increases. (C) BW = +/-100 kHz shows blurred boundary that may occur if BW is too high. Images acquired at 3.0T, 1.2 x 1.2 x 1.2 mm, NEX 4.5, 3:47 min.
C
Figure 6.
Effect of readout bandwidth. Increasing BW can reduce chemical shift artifact and T2 contrast in the background. However, if BW is too high, blurring will occur. (A) BW = +/-62 kHz shows high SNR but with chemical shift artifact. (B) BW = +/-83 kHz shows reduced chemical shift as BW increases. (C) BW = +/-100 kHz shows blurred boundary that may occur if BW is too high. Images acquired at 3.0T, 1.2 x 1.2 x 1.2 mm, NEX 4.5, 3:47 min.
A
Figure 5.
oZTEo images of the pelvis with (A) flip angle of 1 degree and (B) flip angle of 2 degrees; an increase in flip angle increases SNR, however, it also increases T1 contrast in background tissue. Images acquired at 1.5T, 1.25 x 1.25 x 1.3 mm, NEX 4.2, BW = +/-62kHz, 4:00 min.
B
Figure 5.
oZTEo images of the pelvis with (A) flip angle of 1 degree and (B) flip angle of 2 degrees; an increase in flip angle increases SNR, however, it also increases T1 contrast in background tissue. Images acquired at 1.5T, 1.25 x 1.25 x 1.3 mm, NEX 4.2, BW = +/-62kHz, 4:00 min.
A
Figure 7.
The importance of surface coil selection. (A) Posterior array (PA) coil only, (B) PA and anterior array (AA) coil. For MR spine (bone) imaging, the combination of an AA and a PA coil is recommended for the ideal SNR. Images acquired at 3.0T, 1.2 x 1.2 x 1.2 mm, NEX 3.5, BW = +/-62kHz, 3:46 min.
B
Figure 7.
The importance of surface coil selection. (A) Posterior array (PA) coil only, (B) PA and anterior array (AA) coil. For MR spine (bone) imaging, the combination of an AA and a PA coil is recommended for the ideal SNR. Images acquired at 3.0T, 1.2 x 1.2 x 1.2 mm, NEX 3.5, BW = +/-62kHz, 3:46 min.
10. Breighner RE, Endo Y, Konin GP, Gulotta LV, Koff MF, Potter HG. Technical developments: Zero Echo Time Imaging of the Shoulder: Enhanced Osseous Detail by Using MR Imaging. Radiology. 2018 Mar;286(3):960- 966. doi.org/10.1148/radiol.2017170906.
11. de Mello RAF, Ma YJ, Ashir A, et al. Three-Dimensional Zero Echo Time Magnetic Resonance Imaging Versus 3-Dimensional Computed Tomography for Glenoid Bone Assessment. Arthroscopy. 2020 Sep;36(9):2391- 2400. doi.org/10.1016/j.arthro.2020.05.042.
12. Breighner RE, Bogner EA, Lee SC, Koff MF, Potter HG. Evaluation of Osseous Morphology of the Hip Using Zero Echo Time Magnetic Resonance Imaging. Am J Sports Med. 2019 Dec;47(14):3460-3468. doi.org/10.1177/0363546519878170.
9. Carl M, Chiang J-TA. Investigations of the origin of phase differences seen with ultrashort TE imaging of short T2 meniscal tissue. Magn Reson Med. 2012 Apr;67(4):991-1003. doi.org/10.1002/mrm.23075.
13. Argentieri EC, Koff MF, Breighner RE, Endo Y, Shah PH, Sneag DB. Diagnostic Accuracy of Zero-Echo Time MRI for the Evaluation of Cervical Neural Foraminal Stenosis. Spine (Phila PA 1976). 2018 Jul 1;43(13):13, 928-933. doi.org/10.1097/brs.0000000000002462.
14. Cho SB, Baek HJ, Ryu KH, et al. Clinical Feasibility of Zero TE Skull MRI in Patients with Head Trauma in Comparison with CT: A Single-Center Study. AJNR Am J Neuroradiol. 2019 Jan;40(1):109-115. dx.doi.org/10.3174/ajnr.A5916.
15. Lu A, Gorny KR, Ho ML. Zero TE MRI for Craniofacial Bone Imaging. AJNR Am J Neuroradiol. 2019 Sep;40(9):1562-1566. doi.org/10.3174/ajnr.A6175.
16. Delso G, Wiesinger F, Sacolick LI, et al. Clinical evaluation of zero-echo-time MR imaging for the segmentation of the skull. J Nucl Med. 2015 Mar;56(3):417-22. doi.org/10.2967/jnumed.114.149997.
17. Lee C, Jeon KJ, Han SS, et al. CT-like MRI using the zero-TE technique for osseous changes of the TMJ. Dentomaxillofac Radiol. 2020 Mar;49(3):20190272. doi.org/10.1259/dmfr.20190272.
10. Breighner RE, Endo Y, Konin GP, Gulotta LV, Koff MF, Potter HG. Technical developments: Zero Echo Time Imaging of the Shoulder: Enhanced Osseous Detail by Using MR Imaging. Radiology. 2018 Mar;286(3):960- 966. doi.org/10.1148/radiol.2017170906.
11. de Mello RAF, Ma YJ, Ashir A, et al. Three-Dimensional Zero Echo Time Magnetic Resonance Imaging Versus 3-Dimensional Computed Tomography for Glenoid Bone Assessment. Arthroscopy. 2020 Sep;36(9):2391- 2400. doi.org/10.1016/j.arthro.2020.05.042.
12 . Breighner RE, Bogner EA, Lee SC, Koff MF, Potter HG. Evaluation of Osseous Morphology of the Hip Using Zero Echo Time Magnetic Resonance Imaging. Am J Sports Med. 2019 Dec;47(14):3460-3468. doi.org/10.1177/0363546519878170.
18. Xu Y, Shi L, Li N. Value of zero echo time MR imaging and CT in diagnosis of bone destructions of bone tumors and tumor-like lesions. Chinese Journal of Academic Radiology 2020;3:108–114. doi.org/10.1007/s42058-020-00035-1.
‡ Based on 2021 marketing survey including 65 radiologists, radiology directors and chiefs of radiology in five different countries.
10. Breighner RE, Endo Y, Konin GP, Gulotta LV, Koff MF, Potter HG. Technical developments: Zero Echo Time Imaging of the Shoulder: Enhanced Osseous Detail by Using MR Imaging. Radiology. 2018 Mar;286(3):960- 966. doi.org/10.1148/radiol.2017170906.
PREVIOUS
${prev-page}
NEXT
${next-page}
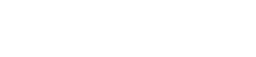
Subscribe Now
Manage Subscription
FOLLOW US
contact us • privacy policy • terms & conditions
© 2023 GE HealthCare. GE is a trademark of the General Electric Company used under trademark license.
result
TECH TRENDS
oZTEo: enabling MR as a one-stop shop for soft tissue and bone imaging
oZTEo: enabling MR as a one-stop shop for soft tissue and bone imaging
by Michael Carl, MR Scientist, Rob Peters, Global Product Marketing Director, Eric Carlson, Clinical Development Specialist MR and PET/MR, Kang Wang, Senior Application Engineer, and Maggie Fung, Global Musculoskeletal MR Development Manager & Applied Science Lab East Leader, GE Healthcare
Introduction
MR imaging has been primarily a soft-tissue imaging modality where tissues such as ligaments, tendons, calcifications and cortical bone structures were assessed from the signal void in the image. Such tissues have extremely short T2 relaxation times because the MR signal has decayed at the time of readout with conventional MR pulse sequences.
Direct MR imaging of short T2 tissues has become possible with novel pulse sequences such as Ultra-Short TE (UTE) or Zero TE (ZTE)1-4. In particular, the prospect of MR bone imaging has gained interest as a way to obtain morphological information about the cortical bone structure in areas such as the shoulder, hip, skull and spine.
Figure 1.
T2 decay of short T2 species such as cortical bone. Conventional imaging technique has readout time that occurs when the signal has significantly decayed. ZTE or UTE imaging are needed to capture these signals.
Clinical benefits of MR bone imaging
In addition to assessing soft tissue contrast in musculoskeletal (MSK) injury, MR can provide complementary cortical bone information that would be co-registered with the soft issue series, making it a one-stop imaging shop for most routine MSK needs. MR bone imaging provides a CT-like image contrast in 3D isotropic resolution without ionizing radiation, making it an attractive alternative for pediatric and pregnant patients who might be otherwise contraindicated for CT. In addition, it provides a welcome alternative when longitudinal CTs are required, particularly when low-dose CT systems are unavailable. In fact, MR bone imaging might also help imaging facilities prioritize their use of CT for more emergent cases. Finally, having both soft tissue assessment and bone imaging within the same exam can lessen the burden on the patient to attend two separate imaging modality appointments and may also lead to reduced healthcare costs.
Different approaches for MR bone imaging
For morphological bone imaging, there have been several methods studied over the past several decades, three of which are described below (Figure 2).
Figure 2.
Comparison of bone imaging using conventional MR, UTE with echo subtraction/IR, and ZTE acquisition. (A) Conventional MR, no signal with long TE to determine bone from void. (B) UTE or ZTE with long T2 suppression using inversion recovery or echo suppression provides direct imaging of the bone, however, with very low SNR. (C) ZTE/UTE demonstrates signal in bone with a flat contrast in the surrounding tissue enabling differentiation between air and soft tissue.
- Dual echo subtraction5,6 Soft tissues and fluid generally have long T2 relaxation times, whereas hard tissues, such as ligaments, tendons and bone have extremely short T2 times. In this method, the first echo is collected at an ultra-short TE time and hence contains signals from both short T2 tissues (e.g., cortical bone) and long-T2 soft tissues. The second echo will only contain signals from the long-T2 soft tissues, while the cortical bone remains mostly dark. When the long and short TE images are subtracted from one another, the long-T2 tissues cancel, and only the short-T2 signals remain. While this technique works well for short-T2 tissues with relatively high proton densities (e.g., meniscus or tendon), the low proton density of cortical bone still makes positive bone contrast challenging, since any residual long-T2 signals that did not fully subtract/cancel (i.e., bone marrow) will compete with the cortical bone signal. This technique effectively requires two scans and is sensitive to motion between acquisitions (Figure 2A).
- Inversion recovery (IR) UTE7 In this technique, the long-T2 tissues are suppressed by the application of a broadband adiabatic inversion pulse, followed by UTE imaging. Adipose and marrow are suppressed by the STIR pulse (TI time at fat’s nullpoint) and muscle (with long T1) is suppressed due to partial nulling and z-magnetization saturation at steady-state. As a result, cortical bone signal can be directly imaged (Figure 2B). However, SNR is usually low in this STIR-type acquisition, which necessitates a long acquisition time for signal averaging. Consequently, this technique is typically not capable of high-resolution imaging and often appears as a low SNR image.
- ZTE imaging using 3D radial acquisition8 The third approach uses a ZTE 3D radial acquisition, which is the basis for oZTEo, and is commercially available in the SIGNA™Works AIR™ IQ Edition software release. ZTE images are inherently low in T2 and T1 contrast, interrupted only by the dark lines representing cortical bone and potentially fascia (Figure 2C). Inverting these images to a reverse scale therefore yields bright signals from the cortical bone and a grey soft-tissue background. This technique has the added benefit of being relatively silent and insensitive to motion, and the sharp contrast between the cortical bone and surrounding soft tissue enables CT-like contrast and is more easily adaptable for segmentation and volume rendering from sub millimeter isotropic resolution.
oZTEo acquisition and optimized reconstruction
In the ZTE acquisition used in oZTEo, the read gradient is ramped-up prior to the excitation and the free induction decay signal is acquired immediately after RF excitation. This allows rapid acquisition efficiency and a near-silent operation due to the lack of rapidly switching gradients. The imaging parameters are also optimized to provide uniform signal intensity (PD contrast) in the soft tissue, such that the cortical bone and calcifications (appears as dark prior to inversion) can be best visualized.
The oZTEo technique is also accompanied with a specialized reconstruction to improve the quality of 3D rendering of these bone images8. The main steps include performing an optimized coil-bias correction algorithm followed by contrast inversion. With a uniform and flat PD-weighted tissue contrast in the background, the volume segmentation can be done with GE Healthcare’s Advantage Workstation (AW) in a few minutes. The new Volume Illumination feature in the AW READYView platform provides realistic cinematic rendering of the bone, which could potentially be helpful in pre-surgical planning and patient/ referring physician education. Once segmented, the AW can also export the result to a standard STL file format for 3D printing if desired (Figure 4).
Figure 4.
(A) Volume rendering using Volume Illumination feature in GE’s AW Volume Viewer. (B) Results can also be exported to common 3D printing formats, such as STL.
oZTEo protocol considerations
For best image quality and optimized volume rendering capability, the ZTE images should be devoid of significant T1 or T2 contrast, or chemical shift artifacts in the long-T2 soft tissues. Isotropic or near isotropic acquisition are also recommended for the best reformat and volume rendering. Here are some parameter optimization approaches that were examined for oZTEo:
- Effect of flip angle on T1 contrast: Increasing the flip angle will increase SNR and T1 contrast. The T1 contrast of a steady-state MR image is mainly determined by the excitation flip angle (FA) in relation to the repetition time (TR). Generally, a larger flip angle or shorter TR results in a more T1-weighted image. In oZTEo, the TR is typically pre-determined by the duration of readout k-space encoding. Therefore, to minimize T1 contrast, one should employ a low flip angle. Figure 5 shows an example of oZTEo comparison between the flip angle of 1 and 2.
- Effect of bandwidth on T2 contrast and chemical shift artifacts: The T2 contrast of an MR image is mainly a function of the sequence echo-time (TE). In oZTEo, this time is near zero, but due to the radial-out k-space acquisition, the T2 has still dependencies on the sequence parameters, such as the readout BW9. Generally, a higher BW results in less T2 contrast, but also yields lower SNR as with conventional MR sequences. In oZTEo, the BW choices are limited to between +/-50-100 kHz, with 62.5 kHz or 83.3 kHz typically used to balance soft tissue contrast, chemical shift artifacts and image sharpness (Figure 6).
- Surface coil intensity correction: For optimal volume rendering results, any non-uniformity due to coil-bias should also be corrected so most of the cortical bone pixels have similar values. oZTEo has been carefully optimized with the coil-bias correction algorithm to improve uniformity of the image.
- Coil selection: oZTEo employs non-selective excitation, therefore, coils should be chosen to provide sufficient FOV coverage without leading to unwanted aliasing artifacts. Specifically for spine, the addition of an anterior coil will help improve vertebrae visualization (Figure 7). oZTEo is compatible with both receive-only and transmit-receive coils. In addition, coils must be designed with sufficient transmit-receive switching time performance, typically with <24 μs, a specification that most GE MR-supported coils can meet.
Figure 5 .
oZTEo images of the pelvis with (A) flip angle of 1 degree and (B) flip angle of 2 degrees; an increase in flip angle increases SNR, however, it also increases T1 contrast in background tissue. Images acquired at 1.5T, 1.25 x 1.25 x 1.3 mm, NEX 4.2, BW = +/-62kHz, 4:00 min.
Figure 6 .
Effect of readout bandwidth. Increasing BW can reduce chemical shift artifact and T2 contrast in the background. However, if BW is too high, blurring will occur. (A) BW = +/-62 kHz shows high SNR but with chemical shift artifact. (B) BW = +/-83 kHz shows reduced chemical shift as BW increases. (C) BW = +/-100 kHz shows blurred boundary that may occur if BW is too high. Images acquired at 3.0T, 1.2 x 1.2 x 1.2 mm, NEX 4.5, 3:47 min.
Figure 7 .
The importance of surface coil selection. (A) Posterior array (PA) coil only, (B) PA and anterior array (AA) coil. For MR spine (bone) imaging, the combination of an AA and a PA coil is recommended for the ideal SNR. Images acquired at 3.0T, 1.2 x 1.2 x 1.2 mm, NEX 3.5, BW = +/-62kHz, 3:46 min.
Early adopter feedback
During development, oZTEo research prototypes were deployed to several GE clinical collaborators for iterative development and feedback within institution review board guidelines. Some of these research studies have led to peer-reviewed publications that generally confirm oZTEo’s clinical value for MR bone imaging, including:
- Bone morphology assessment, e.g., fractures, displaced bone fragments, shoulder instability (Hill-Sachs, Bankart lesion measurements)10,11, hip femoroacetabular impingement (FAI)12, osteonecrosis
- Calcification, e.g., calcific tendinitis, ossification of spine ligaments
- Spinal foraminal stenosis13
- Adult or pediatric skull assessment, e.g., suture closure, facial/head trauma14-16
- Temporomandibular joint (TMJ)17.
These studies demonstrate substantial agreement between CT and MR bone images, both qualitatively and quantitively, in the shoulder, hip, skull, c-spine and TMJ (Table 1). Angular measurements for hip and shoulder have also been compared between MR ZTE and CT with strong agreement10-12. In addition, Breighner, et al. has also found that a majority of the ZTE images provided superior visualization of osseous features compared to standard-of-care MR10, potentially making MR a one-stop-shop for trauma cases where soft tissue and bone morphology need to be accessed together.
Motion robustness is yet another benefit of oZTEo. Since it is based on 3D radial acquisition, it is more motion insensitive compared to Cartesian techniques, especially in c-spine and shoulder applications. The clinical collaborators are also finding new applications for this technique, for example, in the assessment of bone erosions in bone tumors.18
Survey‡ says…
95%
of respondents think that oZTEo MR bone images could lead to a more confident differential diagnosis.
74%
think offering oZTEo will increase their referral volume.
55%
of respondents think oZTEo can benefit pediatric and pregnancy populations.
42%
of respondents said they will add oZTEo to nearly half of their routine exams.
Spine, shoulder and pediatrics are considered the key applications for oZTEo.
Conclusion
Routine MR bone imaging with oZTEo can provide multiple clinical benefits, primarily due to the inherent perfect registration of soft tissue and bony structures. In addition, oZTEo can become a solution for patients for whom radiation exposure from CT is a concern. At present, clinical feedback has been extremely positive, showing strong correlation with CT across multiple different anatomies.
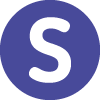