A
Figure 1.
Multi-contrast and multi-planar images acquired with 3D MAGiC prototype sequence. (A, F, K) T2 STIR, (B, G, L) T1w, (C, H, M) double IR, (D, I, N) T1 FLAIR and (E, J, O) conventional T1w.
Images courtesy of Juntendo University Hospital.
B
Figure 1.
Multi-contrast and multi-planar images acquired with 3D MAGiC prototype sequence. (A, F, K) T2 STIR, (B, G, L) T1w, (C, H, M) double IR, (D, I, N) T1 FLAIR and (E, J, O) conventional T1w.
Images courtesy of Juntendo University Hospital.
C
Figure 1.
Multi-contrast and multi-planar images acquired with 3D MAGiC prototype sequence. (A, F, K) T2 STIR, (B, G, L) T1w, (C, H, M) double IR, (D, I, N) T1 FLAIR and (E, J, O) conventional T1w.
Images courtesy of Juntendo University Hospital.
D
Figure 1.
Multi-contrast and multi-planar images acquired with 3D MAGiC prototype sequence. (A, F, K) T2 STIR, (B, G, L) T1w, (C, H, M) double IR, (D, I, N) T1 FLAIR and (E, J, O) conventional T1w.
Images courtesy of Juntendo University Hospital.
E
Figure 1.
Multi-contrast and multi-planar images acquired with 3D MAGiC prototype sequence. (A, F, K) T2 STIR, (B, G, L) T1w, (C, H, M) double IR, (D, I, N) T1 FLAIR and (E, J, O) conventional T1w.
Images courtesy of Juntendo University Hospital.
F
Figure 1.
Multi-contrast and multi-planar images acquired with 3D MAGiC prototype sequence. (A, F, K) T2 STIR, (B, G, L) T1w, (C, H, M) double IR, (D, I, N) T1 FLAIR and (E, J, O) conventional T1w.
Images courtesy of Juntendo University Hospital.
G
Figure 1.
Multi-contrast and multi-planar images acquired with 3D MAGiC prototype sequence. (A, F, K) T2 STIR, (B, G, L) T1w, (C, H, M) double IR, (D, I, N) T1 FLAIR and (E, J, O) conventional T1w.
Images courtesy of Juntendo University Hospital.
H
Figure 1.
Multi-contrast and multi-planar images acquired with 3D MAGiC prototype sequence. (A, F, K) T2 STIR, (B, G, L) T1w, (C, H, M) double IR, (D, I, N) T1 FLAIR and (E, J, O) conventional T1w.
Images courtesy of Juntendo University Hospital.
I
Figure 1.
Multi-contrast and multi-planar images acquired with 3D MAGiC prototype sequence. (A, F, K) T2 STIR, (B, G, L) T1w, (C, H, M) double IR, (D, I, N) T1 FLAIR and (E, J, O) conventional T1w.
Images courtesy of Juntendo University Hospital.
J
Figure 1.
Multi-contrast and multi-planar images acquired with 3D MAGiC prototype sequence. (A, F, K) T2 STIR, (B, G, L) T1w, (C, H, M) double IR, (D, I, N) T1 FLAIR and (E, J, O) conventional T1w.
Images courtesy of Juntendo University Hospital.
K
Figure 1.
Multi-contrast and multi-planar images acquired with 3D MAGiC prototype sequence. (A, F, K) T2 STIR, (B, G, L) T1w, (C, H, M) double IR, (D, I, N) T1 FLAIR and (E, J, O) conventional T1w.
Images courtesy of Juntendo University Hospital.
L
Figure 1.
Multi-contrast and multi-planar images acquired with 3D MAGiC prototype sequence. (A, F, K) T2 STIR, (B, G, L) T1w, (C, H, M) double IR, (D, I, N) T1 FLAIR and (E, J, O) conventional T1w.
Images courtesy of Juntendo University Hospital.
M
Figure 1.
Multi-contrast and multi-planar images acquired with 3D MAGiC prototype sequence. (A, F, K) T2 STIR, (B, G, L) T1w, (C, H, M) double IR, (D, I, N) T1 FLAIR and (E, J, O) conventional T1w.
Images courtesy of Juntendo University Hospital.
N
Figure 1.
Multi-contrast and multi-planar images acquired with 3D MAGiC prototype sequence. (A, F, K) T2 STIR, (B, G, L) T1w, (C, H, M) double IR, (D, I, N) T1 FLAIR and (E, J, O) conventional T1w.
Images courtesy of Juntendo University Hospital.
O
Figure 1.
Multi-contrast and multi-planar images acquired with 3D MAGiC prototype sequence. (A, F, K) T2 STIR, (B, G, L) T1w, (C, H, M) double IR, (D, I, N) T1 FLAIR and (E, J, O) conventional T1w.
Images courtesy of Juntendo University Hospital.
A
Figure 2.
Three-dimensional, high-resolution, simultaneous quantitative mapping of the whole brain with 3D MAGiC demonstrating the accuracy and repeatability of T1, T2 and PD values. (A) T1 map, (B) T2 map and (C) PD map.
Images courtesy of Juntendo University Hospital.
B
Figure 2.
Three-dimensional, high-resolution, simultaneous quantitative mapping of the whole brain with 3D MAGiC demonstrating the accuracy and repeatability of T1, T2 and PD values. (A) T1 map, (B) T2 map and (C) PD map.
Images courtesy of Juntendo University Hospital.
C
Figure 2.
Three-dimensional, high-resolution, simultaneous quantitative mapping of the whole brain with 3D MAGiC demonstrating the accuracy and repeatability of T1, T2 and PD values. (A) T1 map, (B) T2 map and (C) PD map.
Images courtesy of Juntendo University Hospital.
A
Figure 3.
Patient with meningioma scanned with 3D MAGiC prototype, TR/TE=9/4 msec, voxel size=0.5 mm with 1 mm isotropic voxel data acquisition, ARC 2x1, HyperSense factor of 1.5, 7:31 min. (A) Axial T2w, (B) axial T1 FLAIR, (C) axial T1 FLAIR post-contrast, (D) axial T2w, (E) coronal T1 FLAIR and (F) coronal T1 FLAIR post-contrast.
Images courtesy of Juntendo University Hospital.
B
Figure 3.
Patient with meningioma scanned with 3D MAGiC prototype, TR/TE=9/4 msec, voxel size=0.5 mm with 1 mm isotropic voxel data acquisition, ARC 2x1, HyperSense factor of 1.5, 7:31 min. (A) Axial T2w, (B) axial T1 FLAIR, (C) axial T1 FLAIR post-contrast, (D) axial T2w, (E) coronal T1 FLAIR and (F) coronal T1 FLAIR post-contrast.
Images courtesy of Juntendo University Hospital.
C
Figure 3.
Patient with meningioma scanned with 3D MAGiC prototype, TR/TE=9/4 msec, voxel size=0.5 mm with 1 mm isotropic voxel data acquisition, ARC 2x1, HyperSense factor of 1.5, 7:31 min. (A) Axial T2w, (B) axial T1 FLAIR, (C) axial T1 FLAIR post-contrast, (D) axial T2w, (E) coronal T1 FLAIR and (F) coronal T1 FLAIR post-contrast.
Images courtesy of Juntendo University Hospital.
D
Figure 3.
Patient with meningioma scanned with 3D MAGiC prototype, TR/TE=9/4 msec, voxel size=0.5 mm with 1 mm isotropic voxel data acquisition, ARC 2x1, HyperSense factor of 1.5, 7:31 min. (A) Axial T2w, (B) axial T1 FLAIR, (C) axial T1 FLAIR post-contrast, (D) axial T2w, (E) coronal T1 FLAIR and (F) coronal T1 FLAIR post-contrast.
Images courtesy of Juntendo University Hospital.
E
Figure 3.
Patient with meningioma scanned with 3D MAGiC prototype, TR/TE=9/4 msec, voxel size=0.5 mm with 1 mm isotropic voxel data acquisition, ARC 2x1, HyperSense factor of 1.5, 7:31 min. (A) Axial T2w, (B) axial T1 FLAIR, (C) axial T1 FLAIR post-contrast, (D) axial T2w, (E) coronal T1 FLAIR and (F) coronal T1 FLAIR post-contrast.
Images courtesy of Juntendo University Hospital.
F
Figure 3.
Patient with meningioma scanned with 3D MAGiC prototype, TR/TE=9/4 msec, voxel size=0.5 mm with 1 mm isotropic voxel data acquisition, ARC 2x1, HyperSense factor of 1.5, 7:31 min. (A) Axial T2w, (B) axial T1 FLAIR, (C) axial T1 FLAIR post-contrast, (D) axial T2w, (E) coronal T1 FLAIR and (F) coronal T1 FLAIR post-contrast.
Images courtesy of Juntendo University Hospital.
A
Figure 4.
Patient with acoustic tumor scanned with 3D MAGiC protoype, TR/TE=9/4 ms, voxel size=0.5 mm with 1 mm isotropic voxel data acquisition, ARC 2x1, HyperSense factor of 1.5, 7:31 min.
Images courtesy of Juntendo University Hospital.
B
Figure 4.
Patient with acoustic tumor scanned with 3D MAGiC protoype, TR/TE=9/4 ms, voxel size=0.5 mm with 1 mm isotropic voxel data acquisition, ARC 2x1, HyperSense factor of 1.5, 7:31 min.
Images courtesy of Juntendo University Hospital.
C
Figure 4.
Patient with acoustic tumor scanned with 3D MAGiC protoype, TR/TE=9/4 ms, voxel size=0.5 mm with 1 mm isotropic voxel data acquisition, ARC 2x1, HyperSense factor of 1.5, 7:31 min.
Images courtesy of Juntendo University Hospital.
D
Figure 4.
Patient with acoustic tumor scanned with 3D MAGiC protoype, TR/TE=9/4 ms, voxel size=0.5 mm with 1 mm isotropic voxel data acquisition, ARC 2x1, HyperSense factor of 1.5, 7:31 min.
Images courtesy of Juntendo University Hospital.
A
Figure 5.
Patient with pituitary adenoma scanned with 3D MAGiC protoype, TR/TE=9/4 ms, voxel size=0.5 mm with 1 mm isotropic voxel data acquisition, ARC 2x1, HyperSense factor of 1.9, 5:56 min.
Images courtesy of Juntendo University Hospital.
B
Figure 5.
Patient with pituitary adenoma scanned with 3D MAGiC protoype, TR/TE=9/4 ms, voxel size=0.5 mm with 1 mm isotropic voxel data acquisition, ARC 2x1, HyperSense factor of 1.9, 5:56 min.
Images courtesy of Juntendo University Hospital.
C
Figure 5.
Patient with pituitary adenoma scanned with 3D MAGiC protoype, TR/TE=9/4 ms, voxel size=0.5 mm with 1 mm isotropic voxel data acquisition, ARC 2x1, HyperSense factor of 1.9, 5:56 min.
Images courtesy of Juntendo University Hospital.
D
Figure 5.
Patient with pituitary adenoma scanned with 3D MAGiC protoype, TR/TE=9/4 ms, voxel size=0.5 mm with 1 mm isotropic voxel data acquisition, ARC 2x1, HyperSense factor of 1.9, 5:56 min.
Images courtesy of Juntendo University Hospital.
A
Figure 6.
Patient with Sturge-Weber syndrome, scanned with 3D MAGiC protoype, TR/TE=9/4 ms, voxel size=0.5 mm with 1 mm isotropic voxel data acquisition, ARC 2x1, HyperSense factor of 1.9, 5:56 min. (A, D) T2w, (B, E) PSIR and (C, F) T1 map.
Images courtesy of Juntendo University Hospital.
B
Figure 6.
Patient with Sturge-Weber syndrome, scanned with 3D MAGiC protoype, TR/TE=9/4 ms, voxel size=0.5 mm with 1 mm isotropic voxel data acquisition, ARC 2x1, HyperSense factor of 1.9, 5:56 min. (A, D) T2w, (B, E) PSIR and (C, F) T1 map.
Images courtesy of Juntendo University Hospital.
C
Figure 6.
Patient with Sturge-Weber syndrome, scanned with 3D MAGiC protoype, TR/TE=9/4 ms, voxel size=0.5 mm with 1 mm isotropic voxel data acquisition, ARC 2x1, HyperSense factor of 1.9, 5:56 min. (A, D) T2w, (B, E) PSIR and (C, F) T1 map.
Images courtesy of Juntendo University Hospital.
D
Figure 6.
Patient with Sturge-Weber syndrome, scanned with 3D MAGiC protoype, TR/TE=9/4 ms, voxel size=0.5 mm with 1 mm isotropic voxel data acquisition, ARC 2x1, HyperSense factor of 1.9, 5:56 min. (A, D) T2w, (B, E) PSIR and (C, F) T1 map.
Images courtesy of Juntendo University Hospital.
E
Figure 6.
Patient with Sturge-Weber syndrome, scanned with 3D MAGiC protoype, TR/TE=9/4 ms, voxel size=0.5 mm with 1 mm isotropic voxel data acquisition, ARC 2x1, HyperSense factor of 1.9, 5:56 min. (A, D) T2w, (B, E) PSIR and (C, F) T1 map.
Images courtesy of Juntendo University Hospital.
F
Figure 6.
Patient with Sturge-Weber syndrome, scanned with 3D MAGiC protoype, TR/TE=9/4 ms, voxel size=0.5 mm with 1 mm isotropic voxel data acquisition, ARC 2x1, HyperSense factor of 1.9, 5:56 min. (A, D) T2w, (B, E) PSIR and (C, F) T1 map.
Images courtesy of Juntendo University Hospital.
‡Technology in development that represents ongoing research and development efforts. These technologies are not products and may never become products. Not for sale. Not cleared or approved by the US FDA or any other global regulator for commercial availability.
1. Hwang K, Banerjee S, Warntjes M, et al. 3D isotropic multi-parameter mapping and synthetic imaging of the brain with 3D- QALAS: comparison with 2D MAGIC. Proceedings of the 26th Annual Meeting of the International Society for Magnetic Resonance in Medicine, Abstract #5627.
2. Fujita S, Hagiwara A, Hori M, et al. 3D quantitative synthetic MRI-derived cortical thickness and subcortical brain volumes: Scan-rescan repeatability and comparison with conventional T1 -weighted images. J Magn Reson Imaging. 2019;50(6):1834–1842. doi:10.1002/jmri.26744
3. Takei N, Hagiwara A, Fujita S, et al. Accelerated Compressed Sensing 3D multi-parametric imaging Toward Isotropic1mm3 Imaging. Proceedings of the 27th Annual Meeting of the International Society for Magnetic Resonance in Medicine, Abstract #2981.
4. Fujita S, Hagiwara A, Hori M, et al. Accuracy and Repeatability of Three-dimensional Simultaneous Multi-parametric mapping of the Brain based on 3D-QALAS. The 21st Congress of Japan Human Brain Mapping Society, 2019, Abstract #10056.
5. Fujita S, Hagiwara A, Takei N, et al. Compressed sensing applied to 1 mm isotropic multi-parametric imaging with 3D- QALAS: A phantom, volunteer, and patient study. Proceedings of the 28th Annual Meeting of the International Society for Magnetic Resonance in Medicine, Abstract #1877.
A
Figure 1.
Multi-contrast and multi-planar images acquired with 3D MAGiC prototype sequence. (A, F, K) T2 STIR, (B, G, L) T1w, (C, H, M) double IR, (D, I, N) T1 FLAIR and (E, J, O) conventional T1w.
Images courtesy of Juntendo University Hospital.
B
Figure 1.
Multi-contrast and multi-planar images acquired with 3D MAGiC prototype sequence. (A, F, K) T2 STIR, (B, G, L) T1w, (C, H, M) double IR, (D, I, N) T1 FLAIR and (E, J, O) conventional T1w.
Images courtesy of Juntendo University Hospital.
C
Figure 1.
Multi-contrast and multi-planar images acquired with 3D MAGiC prototype sequence. (A, F, K) T2 STIR, (B, G, L) T1w, (C, H, M) double IR, (D, I, N) T1 FLAIR and (E, J, O) conventional T1w.
Images courtesy of Juntendo University Hospital.
D
Figure 1.
Multi-contrast and multi-planar images acquired with 3D MAGiC prototype sequence. (A, F, K) T2 STIR, (B, G, L) T1w, (C, H, M) double IR, (D, I, N) T1 FLAIR and (E, J, O) conventional T1w.
Images courtesy of Juntendo University Hospital.
E
Figure 1.
Multi-contrast and multi-planar images acquired with 3D MAGiC prototype sequence. (A, F, K) T2 STIR, (B, G, L) T1w, (C, H, M) double IR, (D, I, N) T1 FLAIR and (E, J, O) conventional T1w.
Images courtesy of Juntendo University Hospital.
F
Figure 1.
Multi-contrast and multi-planar images acquired with 3D MAGiC prototype sequence. (A, F, K) T2 STIR, (B, G, L) T1w, (C, H, M) double IR, (D, I, N) T1 FLAIR and (E, J, O) conventional T1w.
Images courtesy of Juntendo University Hospital.
G
Figure 1.
Multi-contrast and multi-planar images acquired with 3D MAGiC prototype sequence. (A, F, K) T2 STIR, (B, G, L) T1w, (C, H, M) double IR, (D, I, N) T1 FLAIR and (E, J, O) conventional T1w.
Images courtesy of Juntendo University Hospital.
H
Figure 1.
Multi-contrast and multi-planar images acquired with 3D MAGiC prototype sequence. (A, F, K) T2 STIR, (B, G, L) T1w, (C, H, M) double IR, (D, I, N) T1 FLAIR and (E, J, O) conventional T1w.
Images courtesy of Juntendo University Hospital.
I
Figure 1.
Multi-contrast and multi-planar images acquired with 3D MAGiC prototype sequence. (A, F, K) T2 STIR, (B, G, L) T1w, (C, H, M) double IR, (D, I, N) T1 FLAIR and (E, J, O) conventional T1w.
Images courtesy of Juntendo University Hospital.
J
Figure 1.
Multi-contrast and multi-planar images acquired with 3D MAGiC prototype sequence. (A, F, K) T2 STIR, (B, G, L) T1w, (C, H, M) double IR, (D, I, N) T1 FLAIR and (E, J, O) conventional T1w.
Images courtesy of Juntendo University Hospital.
K
Figure 1.
Multi-contrast and multi-planar images acquired with 3D MAGiC prototype sequence. (A, F, K) T2 STIR, (B, G, L) T1w, (C, H, M) double IR, (D, I, N) T1 FLAIR and (E, J, O) conventional T1w.
Images courtesy of Juntendo University Hospital.
L
Figure 1.
Multi-contrast and multi-planar images acquired with 3D MAGiC prototype sequence. (A, F, K) T2 STIR, (B, G, L) T1w, (C, H, M) double IR, (D, I, N) T1 FLAIR and (E, J, O) conventional T1w.
Images courtesy of Juntendo University Hospital.
M
Figure 1.
Multi-contrast and multi-planar images acquired with 3D MAGiC prototype sequence. (A, F, K) T2 STIR, (B, G, L) T1w, (C, H, M) double IR, (D, I, N) T1 FLAIR and (E, J, O) conventional T1w.
Images courtesy of Juntendo University Hospital.
N
Figure 1.
Multi-contrast and multi-planar images acquired with 3D MAGiC prototype sequence. (A, F, K) T2 STIR, (B, G, L) T1w, (C, H, M) double IR, (D, I, N) T1 FLAIR and (E, J, O) conventional T1w.
Images courtesy of Juntendo University Hospital.
O
Figure 1.
Multi-contrast and multi-planar images acquired with 3D MAGiC prototype sequence. (A, F, K) T2 STIR, (B, G, L) T1w, (C, H, M) double IR, (D, I, N) T1 FLAIR and (E, J, O) conventional T1w.
Images courtesy of Juntendo University Hospital.
result
PREVIOUS
${prev-page}
NEXT
${next-page}
Subscribe Now
Manage Subscription
FOLLOW US
Contact Us • Cookie Preferences • Privacy Policy • California Privacy PolicyDo Not Sell or Share My Personal Information • Terms & Conditions • Security
© 2024 GE HealthCare. GE is a trademark of General Electric Company. Used under trademark license.
TECH TRENDS
A multi-party collaboration in the development of 3D MAGiC for multiparametric imaging
A multi-party collaboration in the development of 3D MAGiC for multiparametric imaging
When MAGiC (MAGnetic resonance image Compilation) was launched by GE Healthcare in 2016, it provided a novel opportunity to capture multiple MR image contrasts in just one scan. However, despite the benefits in clinical efficiency with MAGiC, clinicians are increasingly asking for such a parametric technique in volumetric 3D.
"In our institution, 2D MAGiC has worked nicely for acquiring multiple contrast images," says Shohei Fujita, MD, Department of Radiology, Juntendo University Hospital, Tokyo and Department of Radiology, Graduate School of Medicine, University of Tokyo. "However, in neuro imaging we also want to acquire volumetric data sets."
To address this need, SyntheticMR, the developers of the parameter quantification and synthetic image generation for MAGiC, is partnering with GE Healthcare, Juntendo University and The University of Texas MD Anderson Cancer Center (MDACC) to develop and test 3D MAGiC‡, a new technique that simultaneously maps T1, T2 and proton density (PD) in a single volumetric acquisition.
3D parameter mapping is a challenging problem due to the sheer number of voxels that need to be encoded for fitting spin parameters. The approach by SyntheticMR is based on well established signal models1.
In a 2019 study, synthetic T1 generated from 3D MAGiC was evaluated against a 3D T1-weighted FSPGR sequence and was found to reliably and repeatably measure cortical thickness and subcortical volumes in most brain regions2. At ISMRM 2019, Dr. Fujita copresented a study that demonstrated the use of HyperSense (compressed sensing) with 3D MAGiC maintained image quality and tissue segmentation accuracy3 while benefitting from scan time reduction.
Volumetric, or 3D, imaging is often desired by radiologists to enable advanced visualization with reformatted images in different planes. However, volumetric imaging often comes with a time penalty – it takes longer to acquire the exam than a standard 2D sequence. To address longer scan times, 3D MAGiC is being developed with HyperSense.
"With 3D MAGiC, our customers can expect a quicker MR scanning workflow as compared to standard 3D acquisitions and more data to aid in their diagnosis and treatment monitoring."
Kyle Frye
"In just one 6-minute scan, they will have all the contrasts and image data to perform volume segmentation and quantify the imaging data. We believe it will be a new game-changer in neuro MR imaging," Frye adds.
The 3D acquisition also resolves two issues inherent in the 2D acquisition, which averages spin signal over the slice and sometimes requires slice gaps, both of which negatively impact reformatting in other planes. Thinner, contiguous voxels can be acquired with a 3D acquisition, further expanding potential applications of MAGiC. Using a smaller voxel size will also reduce partial volume effects and potentially provide a more accurate FLAIR image.
Clinical evaluations
Two important clinical areas where 3D MAGiC may have an impact is in oncology and surgical planning.
"Thinner slices and removing the gap between slices provides the potential for clinicians to use 3D MAGiC in oncology or surgical planning sessions," says Frye. "Our clinical partners have been working to validate this expanded use."
Standardization across GE MR scanners will be important in order to compare quantitative values derived from the parametric maps, even when patients are scanned on different scanners at different time points.
Once the sequence is standardized across systems, 3D MAGiC may also potentially simplify clinical protocolling by providing a universal sequence for multiple applications.
Dr. Fujita has been testing the sequence for reliability and repeatability. Using an ISMRM relaxometry phantom, he examined the simultaneous quantitative mapping of T1, T2 and PD in the brain and found them to be as linear and reproducible across scans as a standard MR acquisition method using direct parameter mapping4.
He adds, "We can acquire the same volumetric acquisition as a 3D T1 acquisition and additionally we also have the quantitative T1 and T2 maps with 3D MAGiC. This allows us to calculate the changes for T1, T2 and proton density in small regions to detect subtle changes that may reflect the pathology."
Figure 3.
Patient with meningioma scanned with 3D MAGiC prototype, TR/TE=9/4 msec, voxel size=0.5 mm with 1 mm isotropic voxel data acquisition, ARC 2x1, HyperSense factor of 1.5, 7:31 min. (A) Axial T2w, (B) axial T1 FLAIR, (C) axial T1 FLAIR post-contrast, (D) axial T2w, (E) coronal T1 FLAIR and (F) coronal T1 FLAIR post-contrast.
Images courtesy of Juntendo University Hospital.
Further, radiologists can rely on quantitative data – the measurement tools from 3D MAGiC – rather than rely on subjective interpretation based on what they see in the image.
At Juntendo University, Dr. Fujita has explored the use of 3D MAGiC in pediatrics. In one case of a child with Sturge-Weber syndrome, he also used ARC with a HyperSense (compressed sensing) technique to achieve 1 mm3 iso voxel high spatial resolution within a 6-minute scan time (Figure 6).
"We could clearly see the atrophy in the right hemisphere and the white matter volume loss with the quantitative maps," Dr. Fujita explains. A standard protocol takes nearly 20 minutes, more than three times the length of 3D MAGiC acquisition. Therefore, he sees the potential to rapidly scan pediatric patients and avoid artifacts caused by patient movement that can impact diagnostic image quality.
Dr. Fujita has compared the quantitative values derived from 3D MAGiC with and without HyperSense and has found them to be reliable in either case. In fact, at ISMRM 2020, Dr. Fujita will present a study that enrolled 10 healthy volunteers and 10 multiple sclerosis patients demonstrating the reliability of the volumetric maps from 3D MAGiC using HyperSense5.
As with any 3D sequence, patient movement can impact the reliability of co-registered images. With 3D MAGiC, patient movement can also impact the parametric maps.
"We need a robust approach for patient movement and we think prospective motion correction, or GE’s PROMO, is useful and needed with the sequence," Dr. Fujita adds.
The primary goal of the collaboration between SyntheticMR, GE Healthcare, Juntendo University and MDACC was to address the question of clinical validation and develop a tightly integrated solution that would lead to better adoption for parametric imaging. Extending MAGiC to 3D represents a clear clinical need.
"Bringing together SyntheticMR’s development team with GE’s research and development group and the clinical expertise at Juntendo and MD Anderson has led to a better product," says Ulrik Harrysson, CEO, SyntheticMR. "Working with GE has led to a more tightly integrated solution, providing a seamless operation for the user. Yet, it’s the involvement of the user that is critical for clinical success."
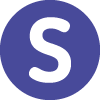