‡Technology in development that represents ongoing research and development efforts. These technologies are not products and may never become products. Not for sale. Not cleared or approved by the US FDA or any other global regulator for commercial availability.
‡Technology in development that represents ongoing research and development efforts. These technologies are not products and may never become products. Not for sale. Not cleared or approved by the US FDA or any other global regulator for commercial availability.
1. American liver foundation. https://liverfoundation.org/for-patients/about-the-liver/diseases-of-the-liver/nonalcoholic-steatohepatitis-information-center/nash-definition-prevalence/.
2. Younossi Z, Tacke F, Arrese M, et al. Global Perspectives on Nonalcoholic Fatty Liver Disease and Nonalcoholic Steatohepatitis. Hepatology. 2019;69(6):2672–2682.
1. American liver foundation. https://liverfoundation.org/for-patients/about-the-liver/diseases-of-the-liver/nonalcoholic-steatohepatitis-information-center/nash-definition-prevalence/.
3Shetty A, Syn WK. Health and Economic Burden of Nonalcoholic Fatty Liver Disease in the United States and Its Impact on Veterans. Fed Pract. 2019;36(1):14–19.
‡Technology in development that represents ongoing research and development efforts. These technologies are not products and may never become products. Not for sale. Not cleared or approved by the US FDA or any other global regulator for commercial availability.
4. Confounder-Corrected Quantitative MRI Biomarkers of Hepatic Iron Content. Available at:
https://clinicaltrials.gov/ct2/show/NCT02025543.
A
Figure 1.
(A) Liver R2*=309 s(-1), (B) liver PDFF=38 percent obtained at 3.0T on a patient with liver iron overload and steatosis.
B
Figure 1.
(A) Liver R2*=309 s(-1), (B) liver PDFF=38 percent obtained at 3.0T on a patient with liver iron overload and steatosis.
A
Figure 2.
(A-D) R2* maps and (E-H) maps of liver iron concentration for (A, C, E, G) a patient with moderate iron overload and (B, D, F, H) a patient with severe iron overload.
B
Figure 2.
(A-D) R2* maps and (E-H) maps of liver iron concentration for (A, C, E, G) a patient with moderate iron overload and (B, D, F, H) a patient with severe iron overload.
C
Figure 2.
(A-D) R2* maps and (E-H) maps of liver iron concentration for (A, C, E, G) a patient with moderate iron overload and (B, D, F, H) a patient with severe iron overload.
E
Figure 2.
(A-D) R2* maps and (E-H) maps of liver iron concentration for (A, C, E, G) a patient with moderate iron overload and (B, D, F, H) a patient with severe iron overload.
F
Figure 2.
(A-D) R2* maps and (E-H) maps of liver iron concentration for (A, C, E, G) a patient with moderate iron overload and (B, D, F, H) a patient with severe iron overload.
G
Figure 2.
(A-D) R2* maps and (E-H) maps of liver iron concentration for (A, C, E, G) a patient with moderate iron overload and (B, D, F, H) a patient with severe iron overload.
H
Figure 2.
(A-D) R2* maps and (E-H) maps of liver iron concentration for (A, C, E, G) a patient with moderate iron overload and (B, D, F, H) a patient with severe iron overload.
D
Figure 2.
(A-D) R2* maps and (E-H) maps of liver iron concentration for (A, C, E, G) a patient with moderate iron overload and (B, D, F, H) a patient with severe iron overload.
5Pooler BD, Hernando D, Reeder SB. Clinical Implementation of a Focused MRI Protocol for Hepatic Fat and Iron Quantification [published online ahead of print, 2019 Mar 27]. AJR Am J Roentgenol. 2019;1–6.
‡Technology in development that represents ongoing research and development efforts. These technologies are not products and may never become products. Not for sale. Not cleared or approved by the US FDA or any other global regulator for commercial availability.
result
PREVIOUS
${prev-page}
NEXT
${next-page}
Subscribe Now
Manage Subscription
FOLLOW US
contact us • privacy policy • terms & conditions
© 2023 GE HealthCare. GE is a trademark of the General Electric Company used under trademark license.
TECH TRENDS
Quick and reproducible liver iron concentration measurements with MR
Quick and reproducible liver iron concentration measurements with MR
Liver disease is a growing global healthcare problem. IDEAL IQ was developed to provide reliable and repeatable fat fraction assessments of the liver. It may also be a useful measurement for liver iron concentration, another precursor for liver disease. Researchers led by Dr. Scott Reeder at the University of Wisconsin Madison are investigating the clinical utility of a modified IDEAL IQ MR sequence‡ to provide the information clinicians need to address this important health issue.
Liver disease is a growing global healthcare problem. IDEAL IQ was developed to provide reliable and repeatable fat fraction assessments of the liver. It may also be a useful measurement for liver iron concentration, another precursor for liver disease. Researchers led by Dr. Scott Reeder at the University of Wisconsin Madison are investigating the clinical utility of a modified IDEAL IQ MR sequence‡ to provide the information clinicians need to address this important health issue.
In 2011, GE Healthcare introduced IDEAL IQ, a non-invasive, fat fraction assessment of the liver. Scott Reeder, MD, PhD, Professor, H.I. Romnes Faculty Fellow, Vice Chair of Research and Chief of MRI at the University of Wisconsin School of Medicine and Public Health in Madison, and his group helped develop IDEAL IQ and its predecessor, IDEAL, a 3-point Dixon technique that provides in-phase, out-of-phase, fat-only and water-only images. It was through this earlier work that Dr. Reeder and Diego Hernando, PhD, Assistant Professor and Director of Quantitative Body MRI at the University of Wisconsin School of Medicine and Public Health, saw the opportunity to utilize a method like IDEAL IQ to quantitatively measure liver iron concentration (LIC) using R2∗ with MR imaging‡.
According to Dr. Reeder, cancer survivors, patients undergoing blood transfusions and people afflicted with thalassemia and sickle cell anemia are also at risk for high LIC. Further, iron overload is also the sine qua non feature of hemochromatosis, a genetic condition that leads to excessive absorption of iron from the gut. In addition to being a precursor for liver disease, too much iron in the body can also lead to iron overload cardiomyopathy, growth and other hormonal disturbances, and in extreme cases, even type 1 diabetes.
"Both iron and fat quantification are clinically relevant in these patient populations," Dr. Reeder explains. For example, adolescents who undergo treatment for leukemia will receive multiple blood transfusions, which leads to iron overload. They, and other cancer survivors, often gain weight during recovery and obesity is associated with the deposition of fat in the liver.
"A fat-corrected iron measurement is important for an accurate measurement of iron," explains Dr. Reeder. "It is also important to have an iron-corrected fat measurement for an accurate measurement of fat."
During the development of IDEAL IQ, Dr. Reeder and Dr. Hernando knew that they would need to measure and correct for R2∗ (=1/T2∗) signal decay.
"IDEAL IQ gives us a fat-corrected R2∗ map, and while that was not the original intent it became very apparent we could use R2∗ to measure liver iron."
Dr. Scott Reeder
Liver disease is a growing worldwide healthcare problem, with non-alcoholic fatty liver disease (NAFLD) affecting 25 percent of the world’s population1,2, of which 20 percent will develop the more aggressive form, nonalcoholic steatohepatitis (NASH)1. As NAFLD progresses, it leads to liver injury, inflammation, fibrosis, cirrhosis and ultimately liver failure. As a result, experts estimate that upwards of 3 million people in the US alone will have cirrhosis by 20303 leading to a significant increase in hepatocellular carcinoma and a growing need for liver transplantation. Hepatic steatosis, the earliest feature of NAFLD, is also associated with cardiovascular disease, the most important morbidity associated with NAFLD. There is also a strong relationship between steatosis, liver fat and the presence of metabolic syndrome. In patients with chronic liver disease including NAFLD, NASH, alcoholic liver disease and hepatitis C viral infection, higher iron levels may also be present.
The presence of fat in the tissue will confound the R2∗ measurement, explains Dr. Hernando, and therefore there is an inherent advantage in terms of accuracy and reproducibility to separate water and fat signals. Several patients who participated in research studies at the UW-Madison had both elevated fat and iron in the liver, further highlighting the need to quantify both, he adds.
The technique
When using IDEAL IQ to quantify fat, the acquisition separates water and fat signals by acquiring multiple echo times where the fat and water signals are acquired in different relative phases.
"In order to provide both fat and iron quantification from the same acquisition, you need to keep the flip angle small to avoid T1 bias," Dr. Hernando says. "If you are only quantifying R2∗, you don’t need to minimize T1 bias in the acquisition. For instance, one can use something closer to the Ernst angle and obtain higher SNR, although the resulting fat quantification would then have some bias."
In the presence of high iron concentration, the R2∗ relaxation rate of the MR signal becomes very large so the signal dissipates very quickly. Therefore, there is an advantage to acquiring shorter echo times in R2∗ acquisitions than would typically be used for measuring fat in patients with iron overload.
Dr. Hernando is researching the use of a modified IDEAL IQ acquisition for accurate and reproducible R2∗ quantification. Although IDEAL IQ is FDA cleared for measuring R2∗, it may not work as well in patients with high LIC levels, such as cancer survivors and those suffering from thalassemia and sickle cell anemia. At UW-Madison, they were able to demonstrate on GE Healthcare 1.5T and 3.0T MR systems that a confounded-corrected R2∗ mapping method was reproducible across acquisition parameters.
Based on this initial research, Dr. Reeder and Dr. Hernando were awarded an NIH grant for a multi-center, multi-vendor study to evaluate an MR-based confounder-corrected R2∗ mapping reconstruction method‡ as a quantitative imaging biomarker of LIC4.
"Our goal was to develop and validate the next-generation fatcorrected R2∗ mapping techniques using a non-linear reconstruction method that improves the dynamic range of the R2∗ measurement across different platforms and field strengths," says Dr. Reeder. The study also compared the results against FerriScan®, an FDA-cleared measurement of LIC in milligrams of iron per dry gram of liver.
Although it is known that R2∗ and LIC are highly correlated, it was unknown whether R2∗ as a biomarker of iron concentration could be reproducible across different choices of acquisition parameters and systems, adds Dr. Hernando.
"We know that R2∗ in the presence of liver iron will be different at 3.0T than 1.5T because it increases with field strength," Dr. Hernando says. "Our overall goal was to develop R2∗ mapping methods that address the relevant confounding factors – the presence of fat, background magnetic field variations and noise at high iron levels – to attain a reliable measurement of R2∗ that is highly correlated with liver iron concentration and reproducible across acquisition parameters, resolution, echo times, imaging orientation and systems. Reproducibility is at the core of the technical validation of quantitative imaging biomarkers."
The study has since closed and results are still emerging during data analysis. However, it is clear to both Dr. Reeder and Dr. Hernando that the results demonstrate that by accounting for all the relevant factors, they have developed a reproducible measurement of R2∗ that uses shorter echo times, high SNR and a non-linear reconstruction method.
One remaining limitation, however, is the use of Cartesian sequences in these studies. Cartesian acquisitions inherently limit echo times – they can be very short but not ultrashort – and therefore in cases of extreme iron overload the method may still fail.
"The natural extension of this in research is to move to ultra-short echo time radial and other non-Cartesian techniques that inherently allow us to acquire much shorter echo times," adds Dr. Hernando. "That may expand the dynamic range of R2∗ mapping in patients with massive iron overload.
However, the fact we could show the Cartesian techniques are reproducible across a wide range of iron overload is an exciting step forward."
What's next
In addition to demonstrating accuracy and reproducibility, Dr. Reeder is particularly enthused about the shorter acquisition time.
"Compared to older spin echo-based methods, this R2∗ method may provide an accurate measure of iron in the liver in a 10-20 second scan versus a 10-20 minute scan."
Dr. Scott Reeder
"That is important for workflow and the patient experience, especially in children where perhaps we can avoid sedation or anesthesia. It is also more precise than previous spin echo techniques, so we can generate better measurements in a lot less time."
This area of research has also led UW-Madison to develop a focused liver fat/iron quantification MR protocol. It is a three breath-hold protocol consisting of a localizer, IDEAL IQ and a single breath hold T2w SSFSE acquisition5. Patients spend a median time of 6 minutes on the table and Dr. Reeder says they have even scanned children as young as seven years old without sedation.
One other area of research interest that has surfaced from this work is the potential to measure magnetic susceptibility of tissue with IDEAL IQ.
"It turns out we can measure quantitative susceptibility mapping, or QSM‡, from the same acquisitions that we use to measure R2∗," says Dr. Hernando. "So, an extension of these projects is to develop, optimize and validate QSM for liver iron quantification. This is a fascinating topic with exciting research value at this earlier technical development stage."
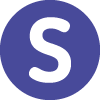